INFLUENCE OF OPTICAL VIBRATIONS ON ENERGY ACTIVATION OF THE CHARGE CARRIER TRAPS AND THE THERMOLUMINESCENCE OF SILICON ORGANIC POLYMER
Сучасне матеріало- та товарознавство :: 1. Актуальні питання наукового та практичного матеріалознавства.
Сторінка 1 з 1
INFLUENCE OF OPTICAL VIBRATIONS ON ENERGY ACTIVATION OF THE CHARGE CARRIER TRAPS AND THE THERMOLUMINESCENCE OF SILICON ORGANIC POLYMER
V. Sugakov1, N Ostapenko2, Yu.Ostapenko2, O. Kerita2,, V.Strelchuk3, O. Kolomys3, A. Watanabe4
1Institute for Nuclear Research, National Academy of Science of Ukraine, 47 Nauki pr., 03680 Kyiv, Ukraine
2Institute of Physics of NASU, pr. Nauky 46, Kiev 03028, Ukraine
3Lashkaryov Institute of Semiconductor Physics of NASU, Nauky 46 pr., Kiev 03028, Ukraine
4Institute of Chemical Reactions, Tohoku University, 980-8578 Sendai, Japan
INFLUENCE OF OPTICAL VIBRATIONS ON ENERGY ACTIVATION OF THE CHARGE CARRIER TRAPS AND THE THERMOLUMINESCENCE OF SILICON ORGANIC POLYMER
Abstract
Energy spectrum of the charge carrier traps was investigated in silicon organic polymer poly(di-n-hexylsilane) by the fractional thermally stimulated luminescence (TSL) in the 5-40 K temperature range. In additional the Raman spectrum of the polymer was also studied at 300 K. For the first time the additional structural features on the TSL curve was observed. It was found that the obtained activation energies of traps coincide with the frequencies of Si-Si vibrations of the polymer chain, active in the Raman spectrum. These results have been explained by the model under which the release of charge carriers from traps may be activated via resonant energy transfer from Si-Si vibrations to the charge carriers. The model explains the appearance of the additional structural features on the TSL curve.
Introduction
Poly(di-n-hexylsilane) (PDHS) belong to silicon organic polymer which consists of -conjugated Si-backbone and hexyl side groups (Fig.1). Due to the delocalization of - bonds in the Si-backbone [1] PDHS has several unique photophysical characteristics, namely: strong absorption in the UV range, high quantum fluorescence yield and high hole mobility. These characteristics determine the possibility of its usage as transport [2] and light-emitting layers [3] in electroluminescent devices. PDHS macromolecule, which consists from the segments of different length, has mainly an ordered trans-conformation at room and lower temperatures. The rotation of the polymer chain segment around the bonds leads to a formation of the conformational defects [1]. These defects create energy barriers for the holes, so that at low temperatures the hole is delocalized within a segment. Thus, segments of different length are the traps for holes. The model of quasi-continuous distribution of traps in polymers [4] is accepted nowadays.
The study of the fractional low temperature thermostimulated luminescence (TSL) provides important information about the presence and the nature of traps and defects for charge carriers. One of the processes determining the properties of TSL is the carriers’ escape from traps. In order to leave a trap and transit to a state with the energy above the mobility threshold a carrier has to acquire additional energy.
The connection between the activation energies of charge carriers traps and the energies of optical vibrations of the polymer backbone observed in the Raman spectra has been recently received in [5] using TSL of PDHS films. It has been proposed a model [6] for the explanation of these results. It was assumed that the processes of a holes release from traps are activated via resonant energy transfer from Si-Si vibrations of the polymer chain that are excited, as the temperature increases, to carriers. The model also predicts the appearance of the additional structure on the TSL curve.
Therefore, we focus herein on detailed correlation between the activation energies of hole traps and the frequencies of Si-Si Raman modes of the polymer chain in the low temperature region of TSL spectra as well as the dependence of the structure in TSL curve. To provide this correlation, it was investigated TSL spectrum of PDHS in the 5 – 40 K and Raman spectrum at 300 K on the same polymer. It was found that TSL curve has fine structure and energy spectrum of traps consists of six horizontal shelves which coincide with the frequencies of Si-Si vibrations of the polymer chain, active in the Raman spectrum. The results are experimental confirmation of the proposed model.
Experimental results. Determination of the activation energy of the charge carrier traps
The fractional TSL measurements were carried out with automatic equipment over a temperature range 5- 40 K with the heating rate of 0.25 K/s. The PDHS films were prepared by direct casting from toluene solution on sapphire substrates. The carriers in the PDHS were photogenerated by the sample excitation by unfiltered light of Hg lamp for 2 min at 5 K.
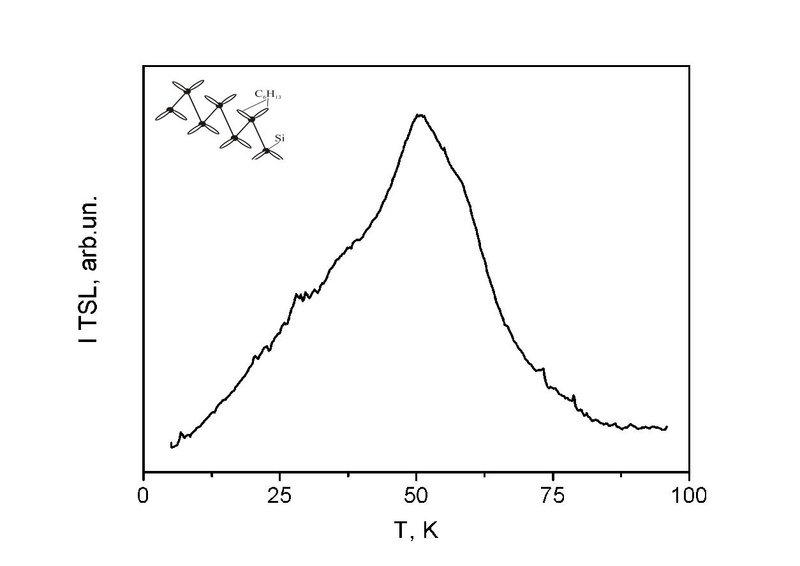
Fig. 1. Integral TSL curve of PDHS film. Inset shows structure of PDHS polymer
. As seen from Fig. 1, the additional structure is clearly observed on the TSL curve. The structure consists of spikes and dips and cannot be considered as a noise because it is repeated in the rerecording.
Fig. 2. Raman spectrum of PDHS powder at 300 K. (Resolution=0.1 cm-1, λex=514.5 nm).
Fig.3 shows the dependence of the activation energy of the traps on the fraction. The numbers on the horizontal shelves are shown the activation energies in eV. The frequencies of Raman spectrum in cm-1 are given in brackets. It is seen that the activation energy traps form six horizontal shelves. Comparison of the data obtained in the study of the TSL and Raman spectra (see Fig.2) showed that the activation energy of the traps correlates well with the frequency of Si-Si vibrations of the polymer chain.
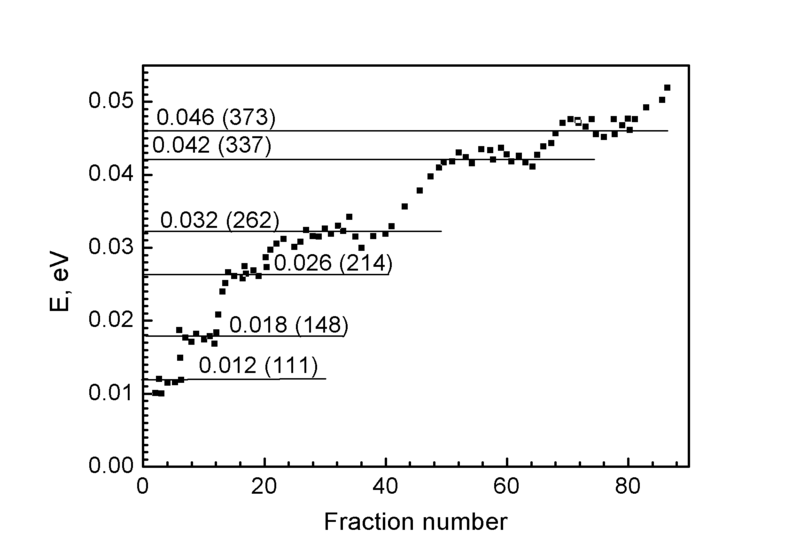
Fig. 3. Dependence of the activation energy of the traps on the fraction number in the 5-40 temperature regions. The numbers on the horizontal shelves are shown the activation energies in eV. The frequencies of Raman spectrum in cm-1 are given in brackets.
This result allows us to conclude, that although the energy spectrum of the traps is the quasi-continuous, the release of the holes in the polymer in proposed model is more probable from those traps, the depth of which corresponds to energy of Si-Si vibration of the polymer chain. Also we detected additional structural features on the TSL curve (Fig.1), which was predicted in presented model in [6]. The suggested in [5] model cannot explain the coincidence of the activation energy with the energy of the vibration. Therefore we use the model suggested in [6], which explained this coincidence and predicted the appearance of the structure.
Base of the model and discussion
The system of the charge carriers captured by traps after irradiation is non-equilibrium. The equilibration in the vibration subsystem happens much faster than the equilibration in the electronic subsystem (in the charge distribution). So, we may suggest that vibration subsystem is in equilibrium state, but the electronic subsystem is nonequilibrium. At such condition there is a process in which energy of the vibration quanta may be transferred to trapped carrier and pulls out it to the state above the mobility threshold. It occurs if localization center has trapped energy coinciding or less than the energy of vibration quantum. Subsequently, the carrier recombines with a charge of opposite sign and manifestoes itself in TSL.
We considered the trapped state dynamics and marked out the processes the charge carries release accompanied by the absorption of vibration quantum separately from all other processes, the probability of which are described by the Arrhenius formula. In this case the time dynamics of charge carrier’s density may be presented in the form

where is the density of localized charges, is the energy of the trapped carrier with respect to the mobility threshold ( ). The term describes the process of localized charge carrier release with the absorption of the vibration quantum. The term describes contribution from the processes involving all other vibrations, including multi-phonon processes, into the processes of charge carrier delocalization.
After solution of the equation (1) we obtain the temperature dependence of the radiation intensity [6]. The analysis shows that in the disordered system 1) the activation energies coincide with the energies of vibration quanta, 2) fine structure arises in the TSL curve in the form of spikes and dips. Therefore, in spite the spectrum of traps in polymer is quasy- continuous, only several of them, energy of activation of which are in resonance with vibration quanta, give studied effect on TSL.
Conclusions
We have studied the energy spectrum of the hole traps of the silicon organic polymer poly(di-n-hexylsilane) by low-temperature fractional TSL method in the 5-40 K temperature range and the Raman spectrum of the polymer at 300 K. A coincidence of the trap activation energy with the frequencies of Si-Si vibrations of the polymer chain, obtained from Raman spectrum, allows us to conclude that the releases of charges in proposed mechanism occur only from those traps, the depth of which corresponds to Si-Si vibrations of the polymer chain. In this region of temperature the additional structural features on the TSL curve is observed, which was predicted in the model [6].
References:
1. M. Pope, C.E. Swenberg, Electronic Processes in Organic Crystals and Polymers (Oxford University Press, N.Y., 1999), p. 877
2. H. Suzuki, H. Meyer, S. Hoshino, D. Haarer, J. Appl. Phys. 78 , 2684 (1995).
3. A. Sharma, M. Katiyar, Deepak, S. Seki and S. Tagawa, Appl. Phys. Lett. 8, 143511 (2006).
4. H. Ba¨ssler, in Semiconducting Polymers: Chemistry, Physics and Engineering, edited by G. Hadziioannou and P. F. van Hutten Wiley-VCH, Weinheim, 2000.
5 A. Gumenyuk, N. Ostapenko, Yu. Ostapenko, O. Kerita, S. Suto. Chem. Phys. 394, 36 (2012).
6. V.I. Sugakov, N.I. Ostapenko, Chem. Phys. 456, 22 (2015).
1Institute for Nuclear Research, National Academy of Science of Ukraine, 47 Nauki pr., 03680 Kyiv, Ukraine
2Institute of Physics of NASU, pr. Nauky 46, Kiev 03028, Ukraine
3Lashkaryov Institute of Semiconductor Physics of NASU, Nauky 46 pr., Kiev 03028, Ukraine
4Institute of Chemical Reactions, Tohoku University, 980-8578 Sendai, Japan
INFLUENCE OF OPTICAL VIBRATIONS ON ENERGY ACTIVATION OF THE CHARGE CARRIER TRAPS AND THE THERMOLUMINESCENCE OF SILICON ORGANIC POLYMER
Abstract
Energy spectrum of the charge carrier traps was investigated in silicon organic polymer poly(di-n-hexylsilane) by the fractional thermally stimulated luminescence (TSL) in the 5-40 K temperature range. In additional the Raman spectrum of the polymer was also studied at 300 K. For the first time the additional structural features on the TSL curve was observed. It was found that the obtained activation energies of traps coincide with the frequencies of Si-Si vibrations of the polymer chain, active in the Raman spectrum. These results have been explained by the model under which the release of charge carriers from traps may be activated via resonant energy transfer from Si-Si vibrations to the charge carriers. The model explains the appearance of the additional structural features on the TSL curve.
Introduction
Poly(di-n-hexylsilane) (PDHS) belong to silicon organic polymer which consists of -conjugated Si-backbone and hexyl side groups (Fig.1). Due to the delocalization of - bonds in the Si-backbone [1] PDHS has several unique photophysical characteristics, namely: strong absorption in the UV range, high quantum fluorescence yield and high hole mobility. These characteristics determine the possibility of its usage as transport [2] and light-emitting layers [3] in electroluminescent devices. PDHS macromolecule, which consists from the segments of different length, has mainly an ordered trans-conformation at room and lower temperatures. The rotation of the polymer chain segment around the bonds leads to a formation of the conformational defects [1]. These defects create energy barriers for the holes, so that at low temperatures the hole is delocalized within a segment. Thus, segments of different length are the traps for holes. The model of quasi-continuous distribution of traps in polymers [4] is accepted nowadays.
The study of the fractional low temperature thermostimulated luminescence (TSL) provides important information about the presence and the nature of traps and defects for charge carriers. One of the processes determining the properties of TSL is the carriers’ escape from traps. In order to leave a trap and transit to a state with the energy above the mobility threshold a carrier has to acquire additional energy.
The connection between the activation energies of charge carriers traps and the energies of optical vibrations of the polymer backbone observed in the Raman spectra has been recently received in [5] using TSL of PDHS films. It has been proposed a model [6] for the explanation of these results. It was assumed that the processes of a holes release from traps are activated via resonant energy transfer from Si-Si vibrations of the polymer chain that are excited, as the temperature increases, to carriers. The model also predicts the appearance of the additional structure on the TSL curve.
Therefore, we focus herein on detailed correlation between the activation energies of hole traps and the frequencies of Si-Si Raman modes of the polymer chain in the low temperature region of TSL spectra as well as the dependence of the structure in TSL curve. To provide this correlation, it was investigated TSL spectrum of PDHS in the 5 – 40 K and Raman spectrum at 300 K on the same polymer. It was found that TSL curve has fine structure and energy spectrum of traps consists of six horizontal shelves which coincide with the frequencies of Si-Si vibrations of the polymer chain, active in the Raman spectrum. The results are experimental confirmation of the proposed model.
Experimental results. Determination of the activation energy of the charge carrier traps
The fractional TSL measurements were carried out with automatic equipment over a temperature range 5- 40 K with the heating rate of 0.25 K/s. The PDHS films were prepared by direct casting from toluene solution on sapphire substrates. The carriers in the PDHS were photogenerated by the sample excitation by unfiltered light of Hg lamp for 2 min at 5 K.
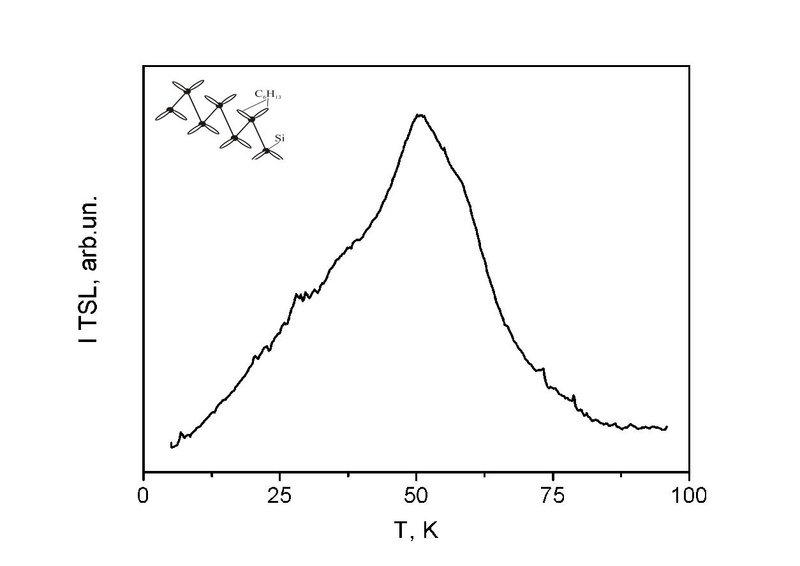
Fig. 1. Integral TSL curve of PDHS film. Inset shows structure of PDHS polymer
. As seen from Fig. 1, the additional structure is clearly observed on the TSL curve. The structure consists of spikes and dips and cannot be considered as a noise because it is repeated in the rerecording.
Fig. 2. Raman spectrum of PDHS powder at 300 K. (Resolution=0.1 cm-1, λex=514.5 nm).
Fig.3 shows the dependence of the activation energy of the traps on the fraction. The numbers on the horizontal shelves are shown the activation energies in eV. The frequencies of Raman spectrum in cm-1 are given in brackets. It is seen that the activation energy traps form six horizontal shelves. Comparison of the data obtained in the study of the TSL and Raman spectra (see Fig.2) showed that the activation energy of the traps correlates well with the frequency of Si-Si vibrations of the polymer chain.
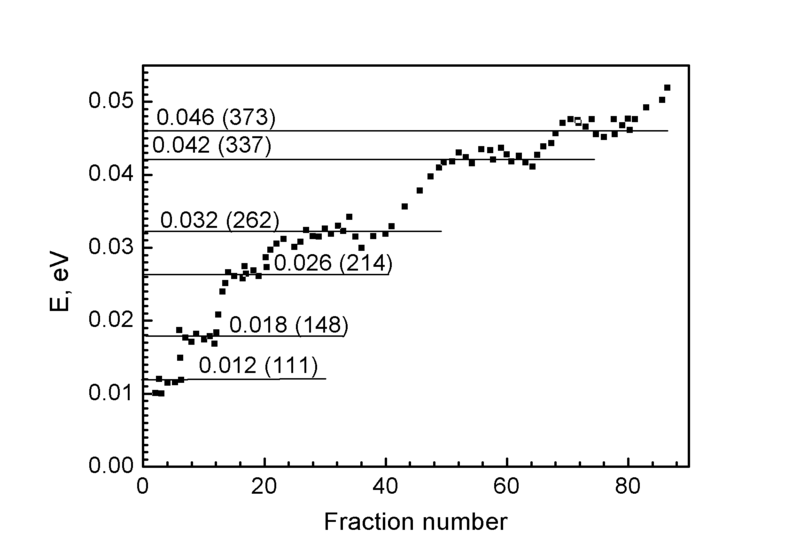
Fig. 3. Dependence of the activation energy of the traps on the fraction number in the 5-40 temperature regions. The numbers on the horizontal shelves are shown the activation energies in eV. The frequencies of Raman spectrum in cm-1 are given in brackets.
This result allows us to conclude, that although the energy spectrum of the traps is the quasi-continuous, the release of the holes in the polymer in proposed model is more probable from those traps, the depth of which corresponds to energy of Si-Si vibration of the polymer chain. Also we detected additional structural features on the TSL curve (Fig.1), which was predicted in presented model in [6]. The suggested in [5] model cannot explain the coincidence of the activation energy with the energy of the vibration. Therefore we use the model suggested in [6], which explained this coincidence and predicted the appearance of the structure.
Base of the model and discussion
The system of the charge carriers captured by traps after irradiation is non-equilibrium. The equilibration in the vibration subsystem happens much faster than the equilibration in the electronic subsystem (in the charge distribution). So, we may suggest that vibration subsystem is in equilibrium state, but the electronic subsystem is nonequilibrium. At such condition there is a process in which energy of the vibration quanta may be transferred to trapped carrier and pulls out it to the state above the mobility threshold. It occurs if localization center has trapped energy coinciding or less than the energy of vibration quantum. Subsequently, the carrier recombines with a charge of opposite sign and manifestoes itself in TSL.
We considered the trapped state dynamics and marked out the processes the charge carries release accompanied by the absorption of vibration quantum separately from all other processes, the probability of which are described by the Arrhenius formula. In this case the time dynamics of charge carrier’s density may be presented in the form

where is the density of localized charges, is the energy of the trapped carrier with respect to the mobility threshold ( ). The term describes the process of localized charge carrier release with the absorption of the vibration quantum. The term describes contribution from the processes involving all other vibrations, including multi-phonon processes, into the processes of charge carrier delocalization.
After solution of the equation (1) we obtain the temperature dependence of the radiation intensity [6]. The analysis shows that in the disordered system 1) the activation energies coincide with the energies of vibration quanta, 2) fine structure arises in the TSL curve in the form of spikes and dips. Therefore, in spite the spectrum of traps in polymer is quasy- continuous, only several of them, energy of activation of which are in resonance with vibration quanta, give studied effect on TSL.
Conclusions
We have studied the energy spectrum of the hole traps of the silicon organic polymer poly(di-n-hexylsilane) by low-temperature fractional TSL method in the 5-40 K temperature range and the Raman spectrum of the polymer at 300 K. A coincidence of the trap activation energy with the frequencies of Si-Si vibrations of the polymer chain, obtained from Raman spectrum, allows us to conclude that the releases of charges in proposed mechanism occur only from those traps, the depth of which corresponds to Si-Si vibrations of the polymer chain. In this region of temperature the additional structural features on the TSL curve is observed, which was predicted in the model [6].
References:
1. M. Pope, C.E. Swenberg, Electronic Processes in Organic Crystals and Polymers (Oxford University Press, N.Y., 1999), p. 877
2. H. Suzuki, H. Meyer, S. Hoshino, D. Haarer, J. Appl. Phys. 78 , 2684 (1995).
3. A. Sharma, M. Katiyar, Deepak, S. Seki and S. Tagawa, Appl. Phys. Lett. 8, 143511 (2006).
4. H. Ba¨ssler, in Semiconducting Polymers: Chemistry, Physics and Engineering, edited by G. Hadziioannou and P. F. van Hutten Wiley-VCH, Weinheim, 2000.
5 A. Gumenyuk, N. Ostapenko, Yu. Ostapenko, O. Kerita, S. Suto. Chem. Phys. 394, 36 (2012).
6. V.I. Sugakov, N.I. Ostapenko, Chem. Phys. 456, 22 (2015).
Сучасне матеріало- та товарознавство :: 1. Актуальні питання наукового та практичного матеріалознавства.
Сторінка 1 з 1
Права доступу до цього форуму
Ви не можете відповідати на теми у цьому форумі